Full Text View
GSA Today
Article, pp. 4-10 | Abstract | PDF (936KB)
Is the “Grenville Front” in the central United States really the Midcontinent Rift?
Cover Image
Table of Contents
- Introduction
- Reevaluating the “Grenville Front” in the U.S.
- The “Front,” The East Arm of the MCR, and Well Data
- Did Deformation near the “Front” Occur during the Grenville Orogeny?
- Grenville-Age Appalachian Inliers and Llano Uplift Compared to Canada
- Discussion
- Summary
- Acknowledgments
- References Cited
Search GoogleScholar for
Search GSA Today

Abstract
Two prominent Precambrian geologic features of central North America are the Midcontinent Rift (MCR) and Grenville Front (GF). The MCR, an extensive band of buried igneous and sedimentary rocks outcropping near Lake Superior, records a major rifting event at ca. 1.1 Ga that failed to split North America. In SE Canada, the GF is the continent-ward extent of deformation of the fold-and-thrust belt from the Grenville orogeny, the sequence of events from ca. 1.3–0.98 Ga culminating in the assembly of the supercontinent of Rodinia. In the central U.S., lineated gravity anomalies extending southward along the trend of the front in Canada have been interpreted as a buried Grenville Front. However, we use recent tectonic concepts and data analyses to argue that these anomalies delineate the eastern arm of the MCR extending from Michigan to Alabama, for multiple reasons: (1) These anomalies are similar to those along the remainder of the MCR and quite different from those across the front in Canada; (2) the Precambrian deformation observed on seismic reflection profiles across the presumed “front” appears quite different from that across the front in Canada, cannot confidently be assigned to the Grenville orogeny, and is recorded at least 100 km west of the “front”; and(3) during the Grenville orogeny, deformational events from Texas to Canada were not caused by the same plate interactions and were not necessarily synchronous. Hence the commonly inferred position of the “Grenville Front” in the central U.S. is part of the MCR, and should not be mapped as a separate entity.
Manuscript received 4 Aug. 2017. Revised manuscript received 13 Oct. 2017. Manuscript accepted 24 Oct. 2017. Posted online 28 Dec. 2017.
© The Geological Society of America, 2017. CC-BY-NC.
Introduction
Two prominent Precambrian geologic features of central North America (Figs. 1 and 2) record different aspects of the Wilson cycle. One, the Midcontinent Rift (MCR), is a U-shaped band of buried igneous and sedimentary rocks that outcrops near Lake Superior. To the south, it is buried by younger sediments, but easily traced because the igneous rocks are dense and highly magnetized (Hinze et al., 1992; Merino et al., 2013). The western arm extends at least to Oklahoma, and perhaps Texas and New Mexico, as evidenced by similar-age diffuse volcanism (Adams and Keller, 1994, 1996; Bright et al., 2014). The eastern arm extends southward through lower Michigan to Alabama (Lyons, 1970; Keller et al., 1982; Dickas et al., 1992; Stein et al., 2014). Although the MCR was often viewed as two arms of a three-arm rifting event in a plate interior, it now appears more likely that it formed as part of the rifting of the Amazonia craton (now in northeastern South America) from Laurentia, the Precambrian core of North America (Stein et al., 2014, 2016). Hence the east and west arms were analogous to the east and west branches of the East African rift, the broad zone forming one arm of the Nubia (west Africa)–Somalia (east Africa)–Arabia three-plate system.
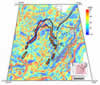
Gravity map showing Midcontinent Rift (MCR), Fort Wayne Rift (FWR), and East Continent Gravity High (ECGH). Grenville-age Appalachian inliers with Laurentia and Amazonia affinities are shown as light and dark gray regions. Grenville Front shown by solid line where observed and dashed line where inferred. OH 1/2 indicates location of COCORP seismic profile (Stein et al., 2014).
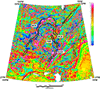
Magnetic anomaly map of the region. Outlines of Midcontinent Rift (MCR), Fort Wayne Rift (FWR), and East Continent Gravity High (ECGH) are from gravity data (Fig. 1). Data source https://pubs.usgs.gov/of/2002/ofr-02-414/ (Bankey et al., 2002).
A second major feature, east of the MCR, is the Grenville Front (GF), also known as the Grenville Front Tectonic Zone. The front is the continent-ward boundary of deformation of the fold-and-thrust belt from the Grenville orogeny, the sequence of orogenic events from ca. 1.3–0.98 Ga culminating in the assembly of the supercontinent of Rodinia (Li et al., 2008) (Fig. 3). Studies in SE Canada, where Grenville rocks are exposed, find that the orogeny involved discrete contractional phases, notably the Shawinigan from ca. 1200–1140 Ma, Ottawan from ca. 1090–1030 Ma, and Rigolet from ca. 1010–980 Ma (Rivers, 2012; McLelland et al., 2013) (Fig. 4A). In SE Canada, erosion has exposed deformed rocks from these orogenic events, from ~54°N to Lake Ontario.
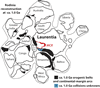
Rodinia reconstruction showing major blocks. After Li et al. (2008). MCR—Midcontinent Rift.
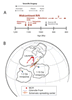
(A) Timeline for evolution of the Midcontinent Rift (MCR) and major phases of the Grenville Orogeny (Malone et al., 2016). (B) Reconstruction of plate positions before Laurentia-Amazonia separation, schematic spreading center geometry, and relevant features (Stein et al., 2014). JBE—Jacobsville Sandstone, Bayfield Group, and other equivalent sandstones.
The orogeny’s phases presumably reflect a series of continental blocks and arcs colliding with and accreting to Laurentia at various locations along its eastern margin. However, the specifics of the plate interactions remain unresolved because the limited paleomagnetic data allow a range of scenarios. In one (Fig. 4B), Amazonia collided with Texas and then moved northward by strike-slip motion relative to Laurentia from ca. 1.18–1.12 Ga (Tohver et al., 2002, 2006). It then rifted from Laurentia, leaving the MCR as a failed third arm, with extension ending ca. 1.096 Ga (Stein et al., 2014, 2015). Amazonia is thought to have recollided with Laurentia somewhat later, causing the Ottawan phase in Canada (McLelland et al., 2013). The southern extent of this collision varies between reconstructions (Li et al., 2008, 2013; Cawood and Pisarevsky, 2017; Merdith et al., 2017).
Even greater uncertainties arise in inferring what occurred during the Grenville orogeny in the U.S. Although the front does not outcrop in the U.S., it has been assumed to extend southward into the U.S. on geological and geophysical grounds. McLaughlin (1954) proposed that it continued through Michigan and Indiana to the New Madrid seismic zone because he considered features such as the Cincinnati Dome to be Grenvillian, which are now considered to be much younger. Bass (1960) suggested that the GF was located to the east, in western Ohio, because data from deep drill holes indicated high-grade metamorphic rocks to the east and unmetamorphosed igneous and sedimentary rocks to the west.
Geophysical data provide the other argument for southward extension of the Grenville Front. In Canada the front is associated with weak gravity and magnetic anomalies (Figs. 1 and 2). Zietz et al. (1966) noted that the proposed front in Ohio coincided with the eastern edge of magnetic and gravity anomalies. Subsequent studies inferred that the GF extended along the East Continent Gravity High (ECGH) through Kentucky and Tennessee to southwest Alabama (Fig. 1). As a result, the GF is often drawn accordingly, although its position varies (e.g., Whitmeyer and Karlstrom, 2007; Baranoski et al., 2009; Bartholomew and Hatcher, 2010; Stein et al., 2014). The absence of the GF between Alabama and the Grenville-age Llano uplift zone in Texas has been attributed to the front’s being rifted away from Laurentia during the latest Precambrian/Cambrian rifting event (Thomas et al., 2012).
Reevaluating the “Grenville Front” in the U.S.
In this paper we argue that the inferred “Grenville Front” in the central U.S. is part of the MCR, rather than the western edge of deformation from the Grenville orogeny. This interpretation is based on several aspects:
- Gravity anomalies in the “front” are similar to those along the remainder of the MCR and quite different from those across the front in Canada;
- Although seismic reflection data near the presumed “front” show faults and possibly suture zones, this deformation appears quite different from the SE-dipping layered structures at the front in Canada, and need not be Grenville age; and
- The Grenville-age events in the Llano uplift area of Texas and much of the eastern U.S. differ, involved different continental fragments, and may have occurred at different times from those in Canada.
The “Front,” The East Arm of the MCR, and Well Data
The often-assumed southward continuation of the “front” in the U.S. is based on gravity and—to a lesser extent—magnetic lineaments. Hence a key question is whether the gravity anomalies along the Fort Wayne Rift and ECGH are associated with the GF or the east arm of the MCR. If they reflect the front, then its assumed location near southeast Michigan implies that the east arm of the MCR ends there (Cannon et al., 1989). However, the gravity highs along the Fort Wayne Rift and ECGH seem to be the continuation of the east arm (Lyons, 1970; Keller et al., 1982; Dickas et al., 1992; Stein et al., 2014), because they are similar in dimensions and magnitude to those elsewhere along the MCR (Figs. 1 and 5) in showing a distinct central high. No similar high occurs across the GF in Canada.
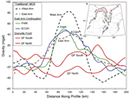
Gravity profiles across Midcontinent Rift (MCR), Fort Wayne Rift (FWR), East Continent Gravity High (ECGH), and Grenville Front (GF) in Canada at locations shown. The gravity highs along the FWR and ECGH seem to be the continuation of the east arm because they are similar in dimensions and magnitude to those elsewhere along the MCR in showing a distinct central high. No similar high occurs across the Grenville Front.
The GF exposed in Canada is severely eroded and represents a deep level of the basal shear zone, not the deformation front observed in modern orogens. The actual deformation front of the Grenville orogen must have been at least several tens of kilometers northwest of the front. As discussed, the gravity highs in the U.S. reflect the MCR’s east arm, so it is unlikely that the GF lay immediately to their east. Shallow-level thrusting of Grenville age would have directly impinged on the recently formed MCR. This seems unlikely given that the gravity data imply that the structure of the MCR’s east arm is similar to that of the west arm, far from any possible GF.
Crustal thickness along the ECGH and FWR is similar to that beneath the MCR. Teleseismic P-wave studies in Tennessee (Owens et al., 1984) show thick crust similar to that beneath the MCR’s west arm (Moidaki et al., 2013; Zhang et al., 2016) and Lake Superior (Green et al., 1989). In southern Ohio, seismic reflection and drilling data support a half-graben structure similar to other parts of the MCR (Dickas et al., 1992). Gravity studies also suggest a thick crust (Keller et al., 1982; Mayhew et al., 1982; Buening, 2013), similar to that on the MCR’s west arm (Merino et al., 2013; Levandowski et al., 2015).
Moreover, new interpretation of the data from wells in Ohio used initially to define the “front” (Bass, 1960) indicates that the geophysical lineament defining the “front” is not a Grenville-age tectonic front but rather part of the MCR, with pre-Grenville Laurentia to the east (Petersson et al., 2015). In Ohio and Kentucky, many of the wells bottom in mafic rocks (Drahovzal et al., 1992; Buening, 2013) similar to MCR rocks exposed near Lake Superior and in the buried west arm (Walker and Misra, 1992; Lidiak, 1996).
Did Deformation near the “Front” Occur during the Grenville Orogeny?
In SE Canada, seismic reflection profiles show parallel southeast-dipping reflectors extending at least 100 km southeastward from the surface trace of the front (Rivers et al., 2012) to at least 20 km depth. However, reflection data across the presumed “front” in the central U.S. look quite different.
Much of the Precambrian tectonic history of Ohio and the “front” is based on the COCORP OH-1 and 2 lines (Fig. 1). Several subsurface features had been interpreted as part of the deformation. In this interpretation, the area to its west was part of the ca. 1.5–1.4 Ga Granite-Rhyolite Province of Laurentia, whereas rocks to the east were similar to provinces of Canada’s exposed Grenville orogen (Culotta et al., 1990). However, recent gravity modeling (Buening, 2013) and analysis of rocks from wells (Petersson et al., 2015) suggest that the Granite-Rhyolite Province continues ~100–150 km eastward beyond the “front,” indicating that this area is not a terrane added during the Grenville Orogeny.
The seismic lines crossing the “front” lack the layered structure seen in Canada, implying a different history. Although they have been interpreted as suggesting Precambrian compression similar to that in Canada, it is unclear whether the compression is of Grenville age. In Canada, Grenville-age metamorphism and shearing of the front are superimposed on rocks recording older events of west-directed thrusting (Bethune, 1997; Rivers et al., 2012). Baranoski et al. (2009) interpreted data at the western end of OH-1 as showing rift development followed by thrust faulting at least ~100 km west of the front.
The inferred age of compressional faulting depends on the age of the faulted Precambrian sedimentary rocks. By default, it has been assumed that the compression must be Grenville in age, so the faulted sediment must be older than 980 Ma (e.g., Drahovzal, 1997). The Proterozoic sandstone Middle Run Formation, observed only in wells, is thought to have similar age to the Jacobsville Sandstone and Bayfield Formation around Lake Superior. The Jacobsville and Middle Run have some similar distributions of Grenville-age zircons. However, detrital zircon dating shows that the Jacobsville must be younger than 959 ± 19 Ma and is probably several hundred million years younger (Malone et al., 2016). Schneider Santos et al.’s (2002) zircon analysis for the Middle Run finds a maximum age of 1048 ± 22 Ma, but they also argue that it must be significantly younger than the Grenville orogeny. If the Jacobsville and Middle Run are about the same age, much of the Middle Run faulting must be younger than Grenville age. These ages show that the “front” in Ohio is not the western edge of the Grenville fold-and- thrust belt and that the deformation near it may be younger, probably reflecting the same post-Jacobsville event that inverted the MCR near Lake Superior (Stein et al., 2015).
Grenville-Age Appalachian Inliers and Llano Uplift Compared to Canada
The argument for a “Grenville Front” in the U.S. assumes that the entire U.S. East Coast was affected by collisions in the Grenville orogeny. Grenville-age events are recorded in Canada and the northeastern U.S. and in exposures in the Llano uplift zone in Texas. It has thus been assumed that similar events occurred between these two areas in the eastern U.S. However, the deformational events in Texas and Canada/NE U.S. were not caused by the same plate collisions and were not necessarily synchronous. Moreover, in the central U.S., although some Grenville-age deformation may have occurred, there is no clear evidence of collisions or of a coherent deformation front, much the less where one has been assumed to be.
Although no Grenville-age fold-and-thrust belt is exposed in the central U.S., Grenville-age features proposed to reflect localized deformation are observed (Ruiz et al., 1984; Bornhorst et al., 1988; Petersson et al., 2015). Most crucially, the Appalachian Mountains in the U.S. contain blocks called Grenville-age Appalachian inliers (GAAI) (Fig. 1). These fragments were assumed to be part of Laurentia during the Grenville orogeny that were later uplifted and exposed at the surface during Paleozoic orogenies (McLelland et al., 2013). These rocks’ ages are usually assigned to phases in the Grenville orogeny (Fig. 4A).
The Llano rocks record compressional events overlapping in time with ones in Canada. However, different plate interactions were involved (Dalziel et al., 2000; Davis and Mosher, 2015). The fact that Grenville-age deformational events were not continuous along Laurentia’s eastern and south margin raises the questions of when the GAAI accreted to Laurentia and whether they record the same events as in Canada.
It seems likely that different tectonic events occurred at different times along the Laurentia margin. Petrologic analyses suggest that GAAI south of about the New Jersey/Pennsylvania border have Amazonian affinity (Fisher et al., 2010; McLelland et al., 2013), implying that they were not part of Laurentia before the Grenville orogeny. In reconstructions of Rodinia (Fig. 3), Amazonia’s southern extent along Laurentia is often near a transition in petrology from northern GAAI with Laurentian affinities to southern ones with Amazonian affinities. Hence, given the Grenville’s complex history and Amazonia’s motion, the history of collisional events in Canada probably does not describe the history of the southern GAAI, especially before the Ottawan phase.
It is worth recognizing the uncertainty in when and how the southern GAAI were sutured to Laurentia. In one interpretation, they were left behind during the 1.1 Ga breakup between Laurentia and Amazonia. Thus, they experienced the two last phases of the Grenville orogeny (Ottawan and Rigolet), assuming collisions in the eastern U.S. were the same as in Canada. Alternatively, they may have collided with Laurentia during the last ~100 m.y. of the Grenville orogeny, but were not on the block that caused deformation in Canada. Another possibility is that the southern GAAI accreted during Rodinia’s breakup. As observed elsewhere, continental fragments can rift off before major breakup (Veevers, 2004). Some GAAI show evidence of rifting and volcanic events starting ca. 760 Ma (McClellan and Gazel, 2014), so if these events are related to others in Laurentia, the southern GAAI accreted to Laurentia before this time. In summary, during the Grenville orogeny the southern GAAI need not have been part of North America. Thus, they cannot with confidence be used to support deformation of the eastern U.S. during the Grenville orogeny (ending ca. 980 Ma).
Grenville deformation south of Amazonia may have involved the Rio de la Plata craton (Fig. 3). Some reconstructions place this block along the southern part of Laurentia’s eastern margin during the Grenville orogeny, but there is much uncertainty about its position owing to the limited paleomagnetic data (Li et al., 2008; Teixeira et al., 2013; Rapalini et al., 2015). Gaucher et al. (2011) argue that detrital zircon distributions of late Neoproterozoic sandstones show that the Rio de la Plata craton was in contact with Laurentia and Amazonia ca. 1 Ga. However, much geographic uncertainty remains, and little is known about the river system distributing these sediments.
Discussion
It seems implausible that the traditionally mapped “Grenville Front” in the central U.S. is the western edge of a Grenville-age fold-and-thrust belt. Recent reinterpretation of well data indicates that this crust was attached to Laurentia before the Grenville orogeny began. Precambrian faulting occurs substantially west of the “front” and may not be of Grenville age. Although some Grenville-age deformation may have occurred in the central U.S., there is no evidence of a coherent front anywhere, much the less where one has been assumed to be. The situation may have been analogous to the isolated pockets of deformation identified in the Midwestern U.S. far inland from the Paleozoic deformation fronts (Marshak et al., 2000; Craddock et al., 2017).
Discarding the “front” makes sense given recent insights into the evolution of the Midcontinent Rift. The “front’s” assumed location near southeast Michigan implies that the east arm of the MCR ended there (Cannon et al., 1989) so rift volcanism and extension did not continue to the east and south. If so, the rift would have been an isolated intraplate event, rather than part of a plate boundary reorganization as implied by paleomagnetic data and similar to those observed today in East Africa and in the geological record elsewhere (Stein et al., 2014).
More precise dating of MCR rocks near Lake Superior than available when the “front” was proposed (McLaughlin, 1954; Bass, 1960) shows that the rift-filling igneous rocks formed ca. 1109–1085 Ma, although rifting started perhaps ~10 m.y. earlier. Seismic reflection data suggest that the extension ended ca. 1096 Ma, ~10 m.y. before basaltic volcanism stopped (Stein et al., 2015). Even if compression occurred near the “front” during the Grenville orogeny, this deformation would be younger than the MCR’s extension. Hence the “front” would not have been there and thus not prevented the rift’s east arm continuing southward.
This timing is consistent with what is known about the initiation of the front in Canada and analogous fronts elsewhere. In a continental collision, deformation starts near the contact and then generally becomes progressively younger toward the interior final front site. Bethune (1997) dates the oldest known GF deformation in Canada at ca. 1035 Ma. Slightly to the east, Rivers (2012) dates the oldest deformation as Ottawan, whereas most metamorphic ages near the front in Canada are from the younger Rigolet phase (Rivers et al., 2012).
The “Grenville Front” issue illustrates the complexity of orogenic belts in space (~5000 km) and time (~300 m.y.). It seems likely that deformational phases varied along Laurentia’s south and east margins. While matching long and fragmented orogenic zones allows reconstructions of the past configurations of continents, orogenic belts with the same age need not be from the same event. A billion years from now, parts of the Andes and Cascadia volcanic arcs might look similar, and the Alpine and Himalayan collisions might appear to have been adjacent.
Summary
New data and insights show that the linear gravity anomalies used to infer the position of the GF in the central U.S. are part of the MCR, and should not be mapped as a separate entity. There is little evidence that this lineation is associated with the western edge of a Grenville fold-and-thrust belt, and good reason to expect that Grenville deformation in the central U.S. would differ from that observed in Canada. It is time to erase the “Grenville Front” lineament in the central U.S. from maps.
Acknowledgments
We thank Scott King, John Weber, and an anonymous reviewer for helpful comments. This work was supported by NSF grants EAR-1550108, EAR-1148088, and EAR-0952345.
References Cited
- Adams, D.C., and Keller, G.R., 1994, Possible extension of the Midcontinental Rift in west Texas and eastern New Mexico: Canadian Journal of Earth Sciences, v. 31, p. 709–720, https://doi.org/10.1139/e94-063.
- Adams, D.C., and Keller, G.R., 1996, Precambrian basement geology of the Permian Basin region of West Texas and eastern New Mexico: A geophysical perspective: The American Association of Petroleum Geologists Bulletin, v. 80, p. 410–431.
- Bankey, V., and 17 others, 2002, Digital data grids for the magnetic anomaly map of North America: U.S. Geological Survey Open-File Report 02-414, https://pubs.usgs.gov/of/2002/ofr-02-414/.
- Baranoski, M.T., Dean, S.L., Wicks, J.L., and Brown, V.M., 2009, Unconformity-bounded seismic reflection sequences define Grenville-age rift system and foreland basins beneath the Phanerozoic in Ohio: Geosphere, v. 5, p. 140–151, https://doi.org/10.1130/GES00202.1.
- Bartholomew, M.J., and Hatcher, R.D., 2010, The Grenville orogenic cycle of southern Laurentia: Unraveling sutures, rifts, and shear zones as potential piercing points for Amazonia: Journal of South American Earth Sciences, v. 29, p. 4–20, https://doi.org/10.1016/j.jsames.2009.08.007.
- Bass, M.N., 1960, Grenville boundary in Ohio: The Journal of Geology, v. 68, p. 673–677, https://doi.org/10.1086/626705.
- Bethune, K.M., 1997, The Sudbury dyke swarm and its bearing on the tectonic development of the Grenville Front: Precambrian Research, v. 85, p. 117–146, https://doi.org/10.1016/S0301-9268(96)00052-6.
- Bornhorst, T.J., Paces, J.B., Grant, N.K., Orbradovich, J.D., and Huber, N.K., 1988, Age of native copper mineralization, Keweenaw Peninsula, Michigan: Economic Geology and the Bulletin of the Society of Economic Geologists, v. 83, p. 619–625, https://doi.org/10.2113/gsecongeo.83.3.619.
- Bright, R.M., Amato, J.M., Denyszyn, S.W., and Ernst, R.E., 2014, U-Pb geochronology of 1.1 Ga diabase in the southwestern United States: Testing models for the origin of a post-Grenville large igneous province: Lithosphere, v. 6, p. 135–156, https://doi.org/10.1130/L335.1.
- Buening, J.D., 2013, Integrated geophysical and geological study of the relationships between the Grenville orogen and mid-continent rift system [M.S. thesis]: Norman, University of Oklahoma, 83 p.
- Cannon, W.F., and 11 others, 1989, The North American Midcontinent Rift beneath Lake Superior from GLIMPCE seismic reflection profiling: Tectonics, v. 8, p. 305–332, https://doi.org/10.1029/TC008i002p00305.
- Cawood, P.A., and Pisarevsky, S.A., 2017, Laurentia-Baltica-Amazonia relations during Rodinia assembly: Precambrian Research, v. 292, p. 386–397, https://doi.org/10.1016/j.precamres.2017.01.031.
- Craddock, J.P., Malone, D.H., Porter, R., Compton, J., Luczaj, J., Konstantinou, A., Day, J.E., and Johnston, S.T., 2017, Paleozoic reactivation structures in the Appalachian-Ouachita-Marathon foreland: Earth-Science Reviews, v. 169, p. 1–34, https://doi.org/10.1016/j.earscirev.2017.04.002.
- Culotta, R.C., Pratt, T., and Oliver, J., 1990, A tale of two sutures: COCORP’s deep seismic surveys of the Grenville province in the eastern U.S. midcontinent: Geology, v. 18, p. 646–649, https://doi.org/10.1130/0091-7613(1990)018<0646:ATOTSC>2.3.CO;2.
- Dalziel, I.W., Mosher, S., and Gahagan, L.M., 2000, Laurentia-Kalahari collision and the assembly of Rodinia: The Journal of Geology, v. 108, p. 499–513, https://doi.org/10.1086/314418.
- Davis, B.R., and Mosher, S., 2015, Complex structural and fluid flow evolution along the Grenville Front, west Texas: Geosphere, v. 11, p. 868–898, https://doi.org/10.1130/GES01098.1.
- Dickas, A.B., Mudrey, M.G., Ojakangas, R.W., and Shrake, D.L., 1992, A possible southeastern extension of the Midcontinent Rift System in Ohio: Tectonics, v. 11, p. 1406–1414, https://doi.org/10.1029/91TC02903.
- Drahovzal, J.A., 1997, Proterozoic sequences and their implications for Precambrian and Cambrian geologic evolution of western Kentucky: Evidence from seismic-reflection data: Seismological Research Letters, v. 68, p. 553–566, https://doi.org/10.1785/gssrl.68.4.553.
- Drahovzal, J.A., Harris, D.C., Wickstrom, L.H., Walker, D., Baranoski, M.T., Keith, B. and Furer, L.C., 1992, The east continent rift basin: A new discovery: Ohio Division of Geological Survey, 28 p.
- Fisher, C.M., Loewy, S.L., Miller, C.F., Berquist, P., Van Schmus, W.R., Hatcher, R.D., Jr., Wooden, J.L., and Fullagar, P.D., 2010, Whole-rock Pb and Sm-Nd isotopic constraints on the growth of southeastern Laurentia during Grenvillian orogenesis: Geological Society of America Bulletin, v. 122, p. 1646–1659, https://doi.org/10.1130/B30116.1.
- Gaucher, C., Frei, R., Chemale, F., Frei, D., Bossi, J., Martínez, G.L., Chiglino, L., and Cernuschi, F., 2011, Mesoproterozoic evolution of the Río de la Plata Craton in Uruguay: At the heart of Rodinia?: International Journal of Earth Sciences, v. 100, p. 273–288, https://doi.org/10.1007/s00531-010-0562-x.
- Green, A.G., Cannon, W.F., Milkereit, B., Hutchinson, D.R., Davidson, A., Behrendt, J.C., Spencer, C., Lee, M.W., Morel-á-LáHuissier, P., and Agena, W.F., 1989, A “GLIMPCE” of the deep crust beneath the Great Lakes, in Mereu, R.F., Mueller, S., and Fountain, D.M., eds., Properties and Processes of Earth’s Lower Crust: American Geophysical Union Geophysical Monograph Series 51, p. 65–80.
- Hinze, W.J., Allen, D.J., Fox, A.J., Sunwood, D., Woelk, T., and Green, A., 1992, Geophysical investigations and crustal structure of the Midcontinent Rift system: Tectonophysics, v. 213, p. 17–32, https://doi.org/10.1016/0040-1951(92)90248-5.
- Keller, G.R., Bland, A.E., and Greenberg, J.K., 1982, Evidence for a major Late Precambrian tectonic event (rifting?) in the eastern Midcontinent region, United States: Tectonics, v. 1, p. 213–223, https://doi.org/10.1029/TC001i002p00213.
- Levandowski, W., Boyd, O.S., Briggs, R.W., and Gold, R.D., 2015, A random-walk algorithm for modeling lithospheric density and the role of body forces in the evolution of the Midcontinent Rift: Geochemistry Geophysics Geosystems, v. 16, p. 4084–4107, https://doi.org/10.1002/2015GC005961.
- Li, Z.X., and 16 others, 2008, Assembly, configuration, and break-up history of Rodinia: A synthesis: Precambrian Research, v. 160, p. 179–210, https://doi.org/10.1016/j.precamres.2007.04.021.
- Li, Z.X., Evans, D.D., and Halverson, G.P., 2013, Neoproterozoic glaciations in a revised global palaeogeography from the breakup of Rodinia to the assembly of Gondwanaland: Sedimentary Geology, v. 294, p. 219–232, https://doi.org/10.1016/j.sedgeo.2013.05.016.
- Lidiak, E.G., 1996, Geochemistry of subsurface Proterozoic rocks in the eastern Midcontinent of the United States: Further evidence for a within-plate tectonic setting, in van der Pluijm, B.A., and Catacosinos, P.A., eds., Basement and Basins of Eastern North America: Geological Society of America Special Paper 308, p. 45–66, https://doi.org/10.1130/0-8137-2308-6.45.
- Lyons, P.L., 1970, Continental and oceanic geophysics, in Johnson, H., and Smith, B.L., eds., The Megatectonics of Continents and Oceans: New Brunswick, New Jersey, Rutgers Press, p. 147–166.
- Malone, D.H., Stein, C.A., Craddock, J.P., Kley, J., Stein, S., and Malone, J.E., 2016, Maximum depositional age of the Neoproterozoic Jacobsville Sandstone: Implications for the evolution of the Midcontinent Rift: Geosphere, v. 12, p. 1271–1282, https://doi.org/10.1130/GES01302.1.
- Marshak, S., Karlstrom, K., and Timmons, J.M., 2000, Inversion of Proterozoic extensional faults: An explanation for the pattern of Laramide and Ancestral Rockies intracratonic deformation, United States: Geology, v. 28, p. 735–738, https://doi.org/10.1130/0091-7613(2000)28<735:IOPEFA>2.0.CO;2.
- Mayhew, M.A., Thomas, H.H., and Wasilewski, P.J., 1982, Satellite and surface geophysical expression of anomalous crustal structure in Kentucky and Tennessee: Earth and Planetary Science Letters, v. 58, p. 395–405, https://doi.org/10.1016/0012-821X(82)90088-7.
- McClellan, E., and Gazel, E., 2014, Cryogenian intra-continental rifting of Rodinia: Evidence from the Laurentian margin in eastern North America: Lithos, v. 206–207, p. 321–337, https://doi.org/10.1016/j.lithos.2014.08.006.
- McLaughlin, D.B., 1954, Suggested extension of the Grenville Orogenic Belt and the Grenville Front: Science, v. 120, p. 287–289, https://doi.org/10.1126/science.120.3112.287.
- McLelland, J.M., Selleck, B.W., and Bickford, M.E., 2013, Tectonic evolution of the Adirondack Mountains and Grenville orogen inliers within the USA: Geoscience Canada, v. 40, p. 318–352, https://doi.org/10.12789/geocanj.2013.40.022.
- Merdith, A., and 11 others, 2017, A full-plate global reconstruction of the Neoproterozoic: Gondwana Research, v. 50, p. 84–134, https://doi.org/10.1016/j.gr.2017.04.001.
- Merino, M., Keller, G.R., Stein, S., and Stein, C., 2013, Variations in Mid-Continent Rift magma volumes consistent with microplate evolution: Geophysical Research Letters, v. 40, p. 1513–1516, https://doi.org/10.1002/grl.50295.
- Moidaki, M., Gao, S.S., Liu, K.H., and Atekwana, E., 2013, Crustal thickness and Moho sharpness beneath the Midcontinent Rift: Reviews of Geophysics, v. 3, p. 1, https://doi.org/10.4081/rg.2013.e1.
- Owens, T.J., Zandt, G., and Taylor, S.R., 1984, Seismic evidence for an ancient rift beneath the Cumberland Plateau, Tennessee: Journal of Geophysical Research, v. 89, p. 7783–7795, https://doi.org/10.1029/JB089iB09p07783.
- Petersson, A., Scherstén, A., Andersson, J., Whitehouse, M.J., and Baranoski, M.T., 2015, Zircon U-Pb, Hf and O isotope constraints on growth versus reworking of continental crust in the subsurface Grenville orogen, Ohio, USA: Precambrian Research, v. 265, p. 313–327, https://doi.org/10.1016/j.precamres.2015.02.016.
- Rapalini, A.E., Tohver, E., Bettucci, L.S., Lossada, A.C., Barcelona, H., and Pérez, C., 2015, The late Neoproterozoic Sierra de las Ánimas Magmatic Complex and Playa Hermosa Formation, southern Uruguay, revisited: Paleogeographic implications of new paleomagnetic and precise geochronologic data: Precambrian Research, v. 259, p. 143–155, https://doi.org/10.1016/j.precamres.2014.11.021.
- Rivers, T., 2012, Upper-crustal orogenic lid and mid-crustal core complexes: Signature of a collapsed orogenic plateau in the hinterland of the Grenville Province: Canadian Journal of Earth Sciences, v. 49, p. 1–42, https://doi.org/10.1139/e11-014.
- Rivers, T., Culshaw, N., Hynes, A., Indares, A., Jamieson, R., and Martignole, J., 2012, The Grenville Orogen—A post–LITHOPROBE perspective, in Percival, J.A., Cook, F.A., and Clowes, R.M., eds., Tectonic Styles in Canada: The LITHOPROBE Perspective: Geological Association of Canada Special Paper 49, p. 97–236.
- Ruiz, J., Jones, L.M., and Kelley, W.C., 1984, Rubidium-strontium dating of ore deposits hosted by Rb-rich rocks, using calcite and other common Sr-bearing minerals: Geology, v. 12, p. 259–262, https://doi.org/10.1130/0091-7613(1984)12<259:RDOODH>2.0.CO;2.
- Schneider Santos, J.O., Hartmann, L.A., McNaughton, N.J., Easton, R.M., Rea, R.G., and Potter, P.E., 2002, Sensitive high resolution ion microprobe detrital zircon geochronology provides new evidence for a hidden Neoproterozoic foreland basin to the Grenville orogen in the eastern Midwest, USA: Canadian Journal of Earth Sciences, v. 39, p. 1505–1515, https://doi.org/10.1139/e02-052.
- Stein, C.A., Stein, S., Merino, M., Keller, G.R., Flesch, L.M., and Jurdy, D.M., 2014, Was the Mid-continent Rift part of a successful seafloor-spreading episode?: Geophysical Research Letters, v. 41, p. 1465–1470, https://doi.org/10.1002/2013GL059176.
- Stein, C.A., Kley, J., Stein, S., Hindle, D., and Keller, G.R., 2015, North America’s Midcontinent Rift: When rift met LIP: Geosphere, v. 11, p. 1607–1616, https://doi.org/10.1130/GES01183.1.
- Stein, S., and 18 others, 2016, When rift met LIP: New insights about the Midcontinent Rift: Eos, v. 97, p. 10–16.
- Teixeira, W., D’Agrella-Filho, M.S., Hamilton, M.A., Ernst, R.E., Girardi, V.A., Mazzucchelli, M., and Bettencourt, J.S., 2013, U–Pb (ID-TIMS) baddeleyite ages and paleomagnetism of 1.79 and 1.59 Ga tholeiitic dyke swarms, and position of the Rio de la Plata Craton within the Columbia supercontinent: Lithos, v. 174, p. 157–174, https://doi.org/10.1016/j.lithos.2012.09.006.
- Thomas, W.A., Tucker, R.D., Astini, R.A., and Denison, R.E., 2012, Ages of pre-rift basement and synrift rocks along the conjugate rift and transform margins of the Argentine Precordillera and Laurentia: Geosphere, v. 8, p. 1366–1383, https://doi.org/10.1130/GES00800.1.
- Tohver, E., van der Pluijm, B.A., van der Voo, R., Rizzotto, R.G., and Scandolara, J.E., 2002, Paleogeography of the Amazon craton at 1.2 Ga: Early Grenvillian collision with the Llano segment of Laurentia: Earth and Planetary Science Letters, v. 199, p. 185–200, https://doi.org/10.1016/S0012-821X(02)00561-7.
- Tohver, E., Teixeira, W., van der Pluijm, B., Geraldes, M.C., Bettencourt, J.S., and Rizzotto, G., 2006, Restored transect across the exhumed Grenville orogen of Laurentia and Amazonia, with implications for crustal architecture: Geology, v. 34, p. 669–672, https://doi.org/10.1130/G22534.1.
- Veevers, J.J., 2004, Gondwanaland from 650–500 Ma assembly through 320 Ma merger in Pangea to 185–100 Ma breakup: Earth-Science Reviews, v. 68, p. 1–132, https://doi.org/10.1016/j.earscirev.2004.05.002.
- Walker, D., and Misra, K.C., 1992, Tectonic significance of basalts of the Middle Run Formation (Upper Proterozoic) of the East Continent Rift Basin, Indiana and Kentucky: Geological Society of America Abstracts with Programs, v. 24, no. 7, p. 330.
- Whitmeyer, S.J., and Karlstrom, K.E., 2007, Tectonic model for the Proterozoic growth of North America: Geosphere, v. 3, p. 220–259, https://doi.org/10.1130/GES00055.1.
- Zhang, H., and 11 others, 2016, Distinct crustal structure of the North American Midcontinent Rift from P wave receiver functions: Journal of Geophysical Research, v. 121, p. 8136–8153.
- Zietz, I., King, E.R., Geddes, W., and Lidiak, E.G., 1966, Crustal study of a continent strip from the Atlantic Ocean to the Rocky Mountains: Geological Society of America Bulletin, v. 77, p. 1427–1448, https://doi.org/10.1130/0016-7606(1966)77(1427:CSOACS)2.0.CO;2.